So far, we've talked about how aromatic ring systems are extra stable due to the delocalization of their pi electrons according to Hückel's rule. Since they're so stable, would they ever want to react with anything at all? Turns out they actually do, but only under certain conditions. However stable they may be, the halo of electron density above and below the ring plane means that aromatic rings are electron rich, and can be made to attack electrophiles and form bonds with them, essentially "substituting" the ring with other groups. The most common type of reaction that you will see involving aromatic compounds is therefore electrophilic aromatic substitution (EAS).
In the late 19th century, Charles Friedel and James Crafts developed a pair of reactions named after them: the Friedel-Crafts alkylation and acylation. Both these reactions are based on EAS, and differ only in the type of functional group added to the aromatic ring. Let's look at the alkylation reaction first. As the name suggests, an alkyl group is being added to the aromatic ring. The source of the alkyl group is an alkyl halide, but if you just mixed the aromatic compound with the alkyl halide, nothing will happen. The aromatic ring is just too weak of a nucleophile to attack the alkyl halide directly in an Sn2-type reaction. We need to add another reagent into the mix, something that will activate the alkyl halide to make it a much stronger electrophile. To this end, we introduce a halogen carrier, such as iron(III) chloride. Halogen carriers are typically very strong Lewis acids, and would rush for any electron lone pairs they find, in this case, a lone pair on the halide atom. This separates the halide atom from the alkyl halide, generating the alkyl carbocation. The aromatic ring can now attack this carbocation, forming a new bond with it.
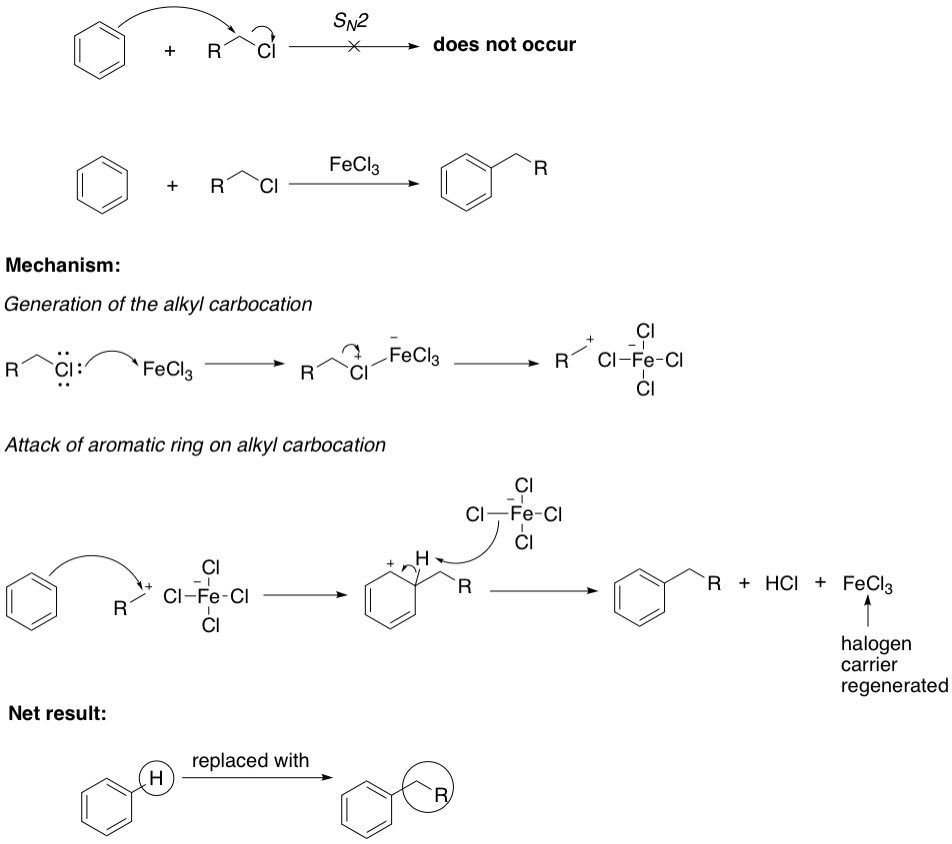
This action breaks the aromaticity of the ring, and we've learnt that molecules don't like that and will do anything to become aromatic again. In this case, the proton on the same carbon that attacked the alkyl carbocation is more than happy to leave as HCl, helped along by the attack of a Cl- provided by the FeCl4- anion. This regenerates the halogen carrier FeCl3, and therefore it functions as a catalyst in this reaction. The net result of this reaction is the substitution of a hydrogen atom in the ring with the alkyl group, hence the name electrophilic aromatic substitution.
The Friedel-Crafts acylation mechanism is identical to the alkylation, but with a different electrophile: the acyl group. In this reaction, an acyl chloride is the source of the acyl group. The halogen carrier is the same, and it removes the chloride to form an acylium ion, the activated acyl source. The ring can then attack the acylium ion, breaking its aromaticity, and an identical process occurs to rearomatize and regenerate the halogen carrier.
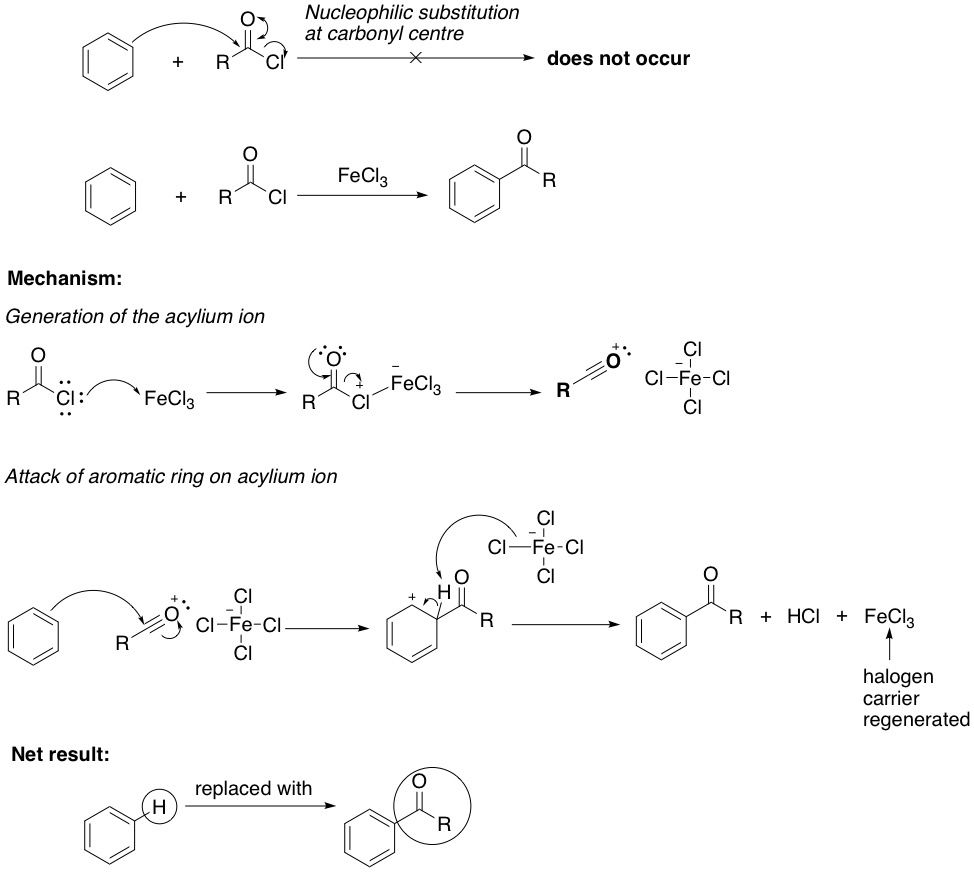
So far we've only looked at benzene, which doesn't contain any substituents. But what if there are already substituents on the ring? Substituents alter the electron density distribution on the ring, making certain carbon atoms have a higher electron density then others and thereby favouring EAS at them. We say that substituents have a directing effect on an aromatic ring. In benzene ring systems, a nomenclature for naming carbon positions relative to a substituent exists. These are the ortho, meta and para positions. Although this nomenclature has been largely superseded by the IUPAC numerical system, it is still widely used by the organic chemistry community because of its convenience. In the example below, we say that the nitro group is ortho to the hydroxyl. Vice versa, the hydroxyl is ortho to the nitro group. We can see that this is a relative nomenclature only.
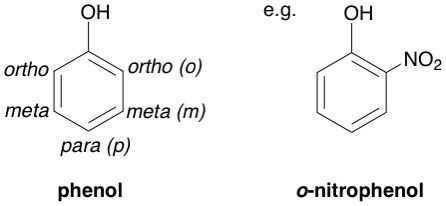
Phenol is a good example of the directing effects of substituents. The oxygen atom in the hydroxyl group contains lone pairs that can delocalize into the ring. For this reason we consider hydroxy or alkoxy (OH, OR) groups to be electron donating. We can draw a series of resonance structures to show this.
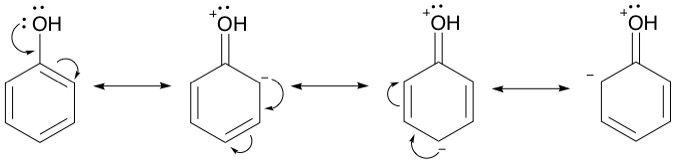
From these resonance structures, we see that the negative charge can rest only on the ortho and para positions. Because of this, EAS is favoured at these positions, and will produce predominantly ortho and para products. Invariably, some meta product will be formed from non-directed attack, but only in insignificant amounts. This trend applies to all substituents that are considered electron donating: alkyl groups (R), hydroxy, alkoxy, amines, halogens are all considered ortho and para directing. Now since these groups increase electron density in the ring especially at the ortho and para positions, the rate of EAS would naturally increase relative to an unsubstituted ring. Therefore, we say that these groups are activating. There are exceptions among the halogens which will be discussed below.
What if we replaced the hydroxyl group with an electron withdrawing group, such as a nitro group? Again, we turn to resonance structures to help us answer this question.
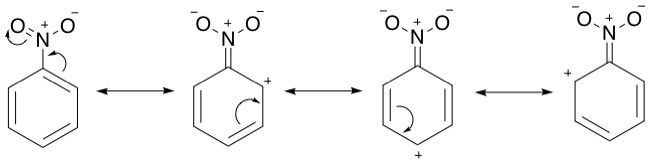
Being one of the strongest electron withdrawing groups, the nitro group pulls electron density from the ring towards itself. Now, the opposite situation from phenol happens: the ortho and para positions have positive charges on them, therefore they are electron deficient and unfavourable for EAS, leaving only the meta position available. Therefore, EAS takes place predominantly at the meta positions when electron withdrawing substituents are present. This trend applies to substituents that are considered electron withdrawing: nitro, acyl, and nitrile groups are considered meta directing. Now since these groups decrease electron density in the ring especially at the ortho and para positions, the rate of EAS would naturally decrease relative to an unsubstituted ring. Therefore, we say that these groups are deactivating.
In summary, electron donating substituents are activating and ortho and para directing, and electron withdrawing substituents are deactivating and meta directing.
The halogen conundrum:
So far we've established the halogens as electron donating substituents that activate an aromatic ring towards ortho and para directed EAS. But aren't the halogens electron withdrawing due to their electronegativity? Yes they are, and fluorine is definitely electron withdrawing under most circumstances because it's the most electronegative element. But bear in mind that when connected to an aromatic ring, the halogens also have lone pairs of electrons that can delocalize into the ring, and fluorine, having its lone pairs in 2p orbitals are of the same size as those of the carbons in the ring, therefore allowing them to overlap much better than say, those of chlorine (lone pairs in larger 3p orbitals), and bromine (lone pairs in much larger 4p orbitals). This effect outweighs the inductive effect caused by fluorine's electronegativity, and therefore we consider fluorine to be electron donating and activating.
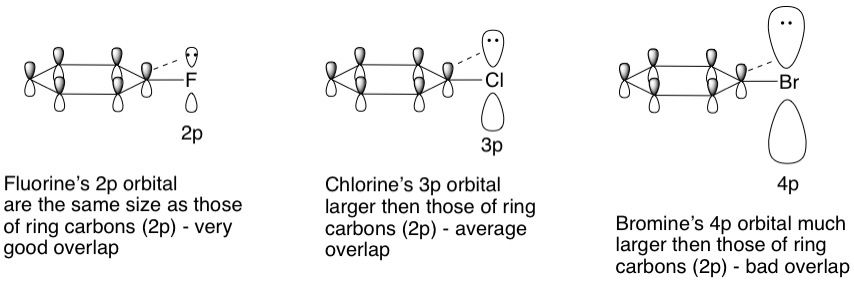
Since the other halogens (Cl, Br and I) can't delocalize their lone pairs as well as fluorine (but there is still some measure of it), inductive effects from their electronegativities become more prominent and therefore they are considered deactivating. But they are still ortho and para directors due to that small measure of lone pair delocalization. This halogen conundrum is sort of like the clutch pedal in a manual transmission car, where that 'sweet spot' causes the car to be in a half-stopped and half-moving state i.e. like these halogens causing the ring to be deactivated, but still behave like it's an activated one.
Comentarios