Aromaticity - Conjugation in circles
- Benjamin Hui
- Feb 3, 2019
- 5 min read
Previously, we discussed how conjugation (double bonds in series) stabilizes a molecule by lowering the HOMO and LUMO energy levels of the molecule. We also looked at the MO diagrams of butadiene and hexatriene. Can conjugation also occur in cyclic compounds? Absolutely. Let's have a look at a classic molecule: Benzene, whose structure was correctly proposed by August Kekulé in 1865. This is a 6-membered ring with three conjugated double bonds. This means that all the carbon atoms in benzene are sp^2-hybridized because each carbon needs to have a free p-orbital to form a pi-bond with its neighbour. This also means that benzene is a flat, or planar molecule because sp^2-hybridized atoms always adopt a flat geometry. Because of this, all six p-orbitals are in perfect alignment on the plane, allowing all of them to overlap and thereby delocalizing the pi electrons.
Delocalization of the pi electrons has a particular effect on the carbon-carbon bonds. The double bonds are no longer pure double bonds. Instead, they become something between a single and double bond, more like 1.5. Similarly, the previously single bonds are no longer pure single bonds. They also become 1.5 bonds. In short, all the C-C bonds become equivalent. This has been experimentally proven with the actual measurement of the C-C bond lengths in benzene: they are all identical and fall between the lengths of a pure double and pure single bond.
Because of the delocalization of the pi electrons around the ring, benzene is often structurally represented by a hexagon with a circle in the middle. However, when we draw mechanisms for reactions involving benzene, we employ the Kekulé structure which shows the double bonds so that we can show the electron flow clearly with curly arrows. Yes, this may be confusing because we've established the lack of absolute double bonds in benzene in reality, but this is purely for the sake of convenience and electron accounting. It's easier to represent benzene reactions on paper if we started from a structure showing clearly where electrons are coming from.
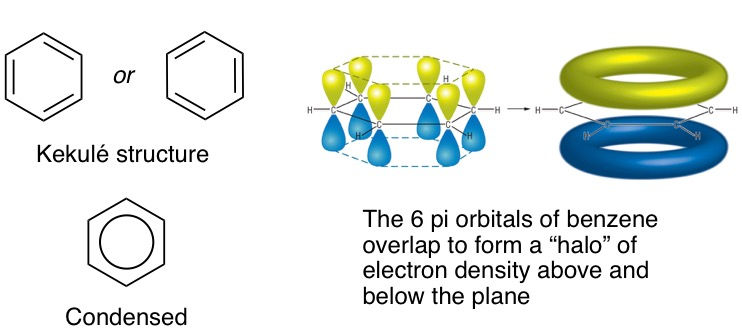
Although benzene does participate in reactions, the most famous being Friedel-Craft reactions (more on this later), its cyclical, conjugated and planar nature (all ring atoms sp^2 hybridized) confers it a special form of stability called aromaticity. In addition, the number of pi electrons is a deciding factor in determining aromaticity. Hückel's rule states that a molecule, in addition to being cyclic, conjugated and planar, needs to have 4n + 2 pi electrons, where n is an integer i.e. 0, 1, 2 etc. Since benzene has 6 pi electrons, n = 1, which satisfies the requirement. An easy way to tell if a molecule is aromatic is to count the number of pi electrons and solve for n. If n is an integer, it is aromatic. If it's a fraction, it's not.
The challenge in most organic chemistry problems about aromaticity is to correctly count the number of pi electrons in the molecule. For most students, counting electrons in a carbocyclic molecule (all ring atoms are carbon) is pretty straightforward. The problem arises when there are heteroatoms in the ring i.e. N, O or S. So let's move on from benzene to look at another molecule: pyridine. This molecule is identical to benzene in all aspects except that one carbon atom has been replaced by a nitrogen. Is pyridine aromatic then? First, we need to identify if the molecule is planar by confirming all ring atoms are sp^2-hybridized. We know for sure the carbon atoms are, but what about the nitrogen? Well, we can see it has a double bond, so it must have a free p-orbital, which makes it sp^2-hybridized. Therefore, pyridine is planar, conjugated, and like benzene, has 6 pi electrons, making it aromatic.
Let's change the molecule a bit by hydrogenating it. We now have a compound called 1,2-dihydropyridine. Is this molecule aromatic? Well, let's check if all the ring atoms are sp^2-hybridized. We see that the nitrogen and an adjacent carbon no longer have double bonds. This makes both of them sp^3-hybridized. This condition alone renders the molecule non-aromatic.
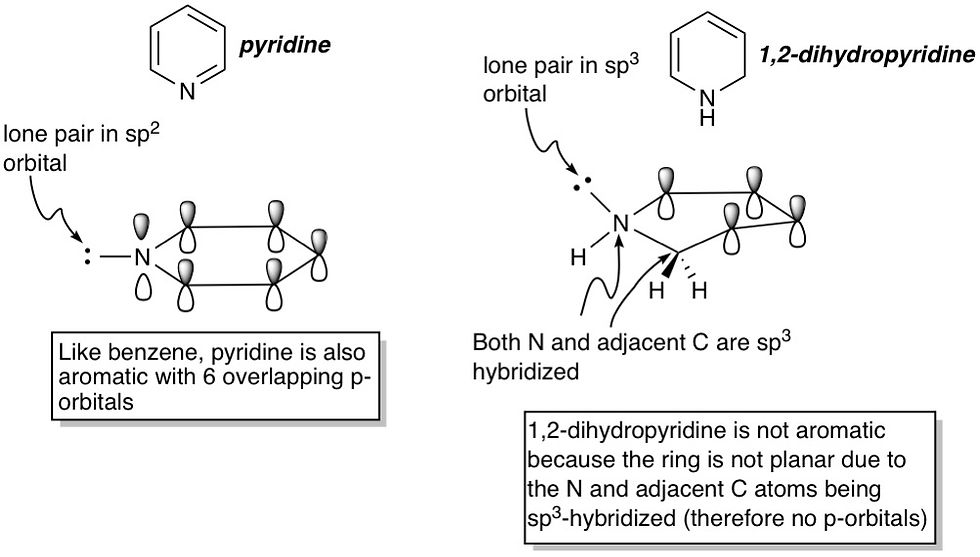
Now, we'll up the ante a little by reducing the ring size, while retaining a heteroatom. Pyrrole is a 5-membered ring with a nitrogen. The four carbon atoms are all sp^2-hybridized because they all have double bonds, so they contribute a total of 4 pi electrons. The nitrogen doesn't have any double bonds, so by all considerations it should be sp^3-hybridized, and the molecule shouldn't be aromatic because it wouldn't be planar and n works out to be 0.5 by Hückel's rule. But here's the surprise: Pyrrole actually IS aromatic. How though? Well, aromaticity offers a cyclic conjugated molecule such a great stability boost that it would always try to become aromatic if it can. For pyrrole, this means that the nitrogen rehybridizes itself to become sp^2, thereby forcing its lone pair into a p-orbital. This p-orbital, with its two electrons, can now contribute to the pi system, making it 6 electrons total and therefore aromatic. This phenomenon occurs in the oxygen and sulfur analogs of pyrrole as well: furan and thiophene respectively, as well as other fused-ring systems containing these 5-membered fragments such as indole, benzofuran and benzothiophene. As an exercise, try counting the number of pi electrons in these fused systems and applying Hückel's rule to verify their aromaticity. You should get 10 electrons with n = 2.
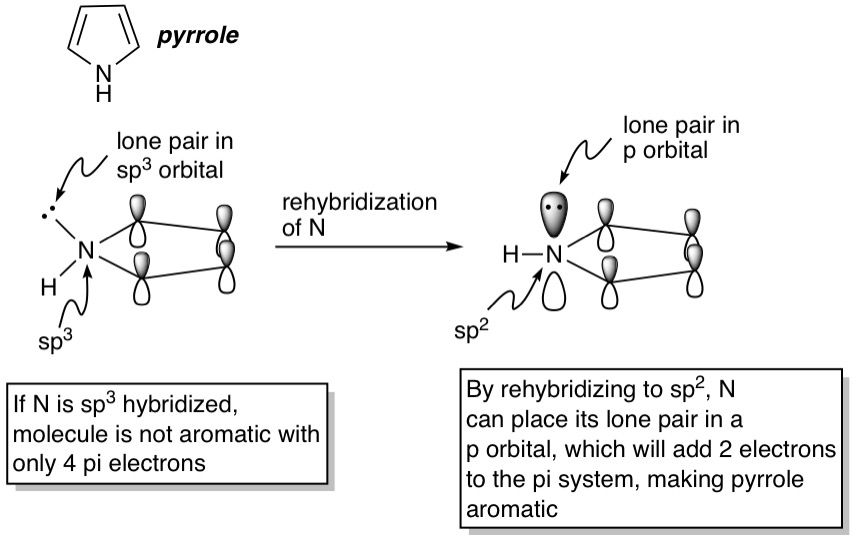
If you're familiar with the ARIO priority system for comparing compound acidities (I've posted an article on this), you'll know that the A stands for Aromaticity, and should be the first thing you look for when asked to compare compound acidities or explain observed pKas. If a compound can become aromatic by releasing a proton, it would be super acidic compared to a similarly structured compound that doesn't become aromatic on deprotonation. An example is that of cyclopentadiene (pKa = 15) and cyclopentane (pKa = 50). Cyclopentadiene itself is not aromatic. It only has 4 pi electrons and is non-planar due to the sp^3 carbon. However, when it loses a proton, the resultant lone pair is forced into a p-orbital much like what happens in pyrrole, giving us 6 pi electrons and making the molecule aromatic. Cyclopentane, on the other hand, doesn't have any pi electrons to begin with because all the carbons are sp^3 hybridized and so has no reason to lose a proton. This is a dramatic example of how aromaticity can effect the acidity of compounds: The pKa difference of 35 between cyclopentadiene and cyclopentane translates to cyclopentadiene being 10^35 times more acidic! That's an unfathomably huge number.
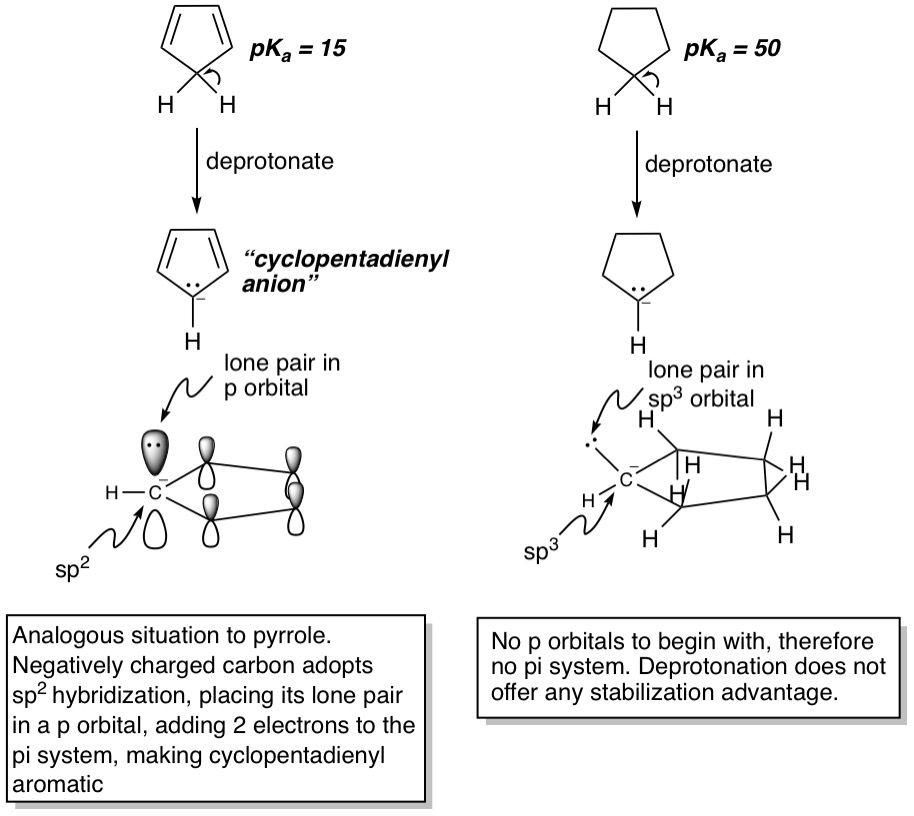
Instead of the pre-aromatic compound losing a proton to become an aromatic negatively charged conjugate base, the opposite can also occur, where a leaving group leaves to form an aromatic, positively charged carbocation. Take the compound below for example, which contains 7 carbon atoms in a ring. The bromine-bound one is sp^3 hybridized, so this molecule is not aromatic. However, if the Br leaves, the carbon becomes positively charged, identical to the first step of an Sn1 reaction. This transforms it into sp^2 hybridization, as carbocations always are, leaving an empty p-orbital which is then free to allow electrons to delocalize into it. We can think of this empty p-orbital as "completing the circuit", allowing electrons to circulate freely in this cyclic system. In other words, every ring atom in an aromatic system needs to possess a p-orbital, empty or occupied. This is just another way of saying that every ring atom in an aromatic system needs to be sp^2 hybridized.
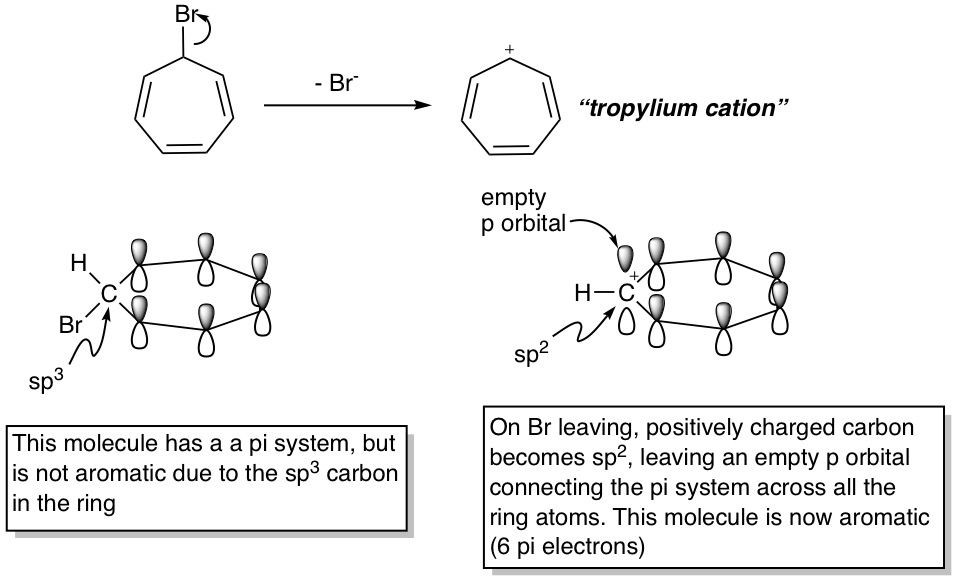
Here are some practice problems, and the solutions can be found here:
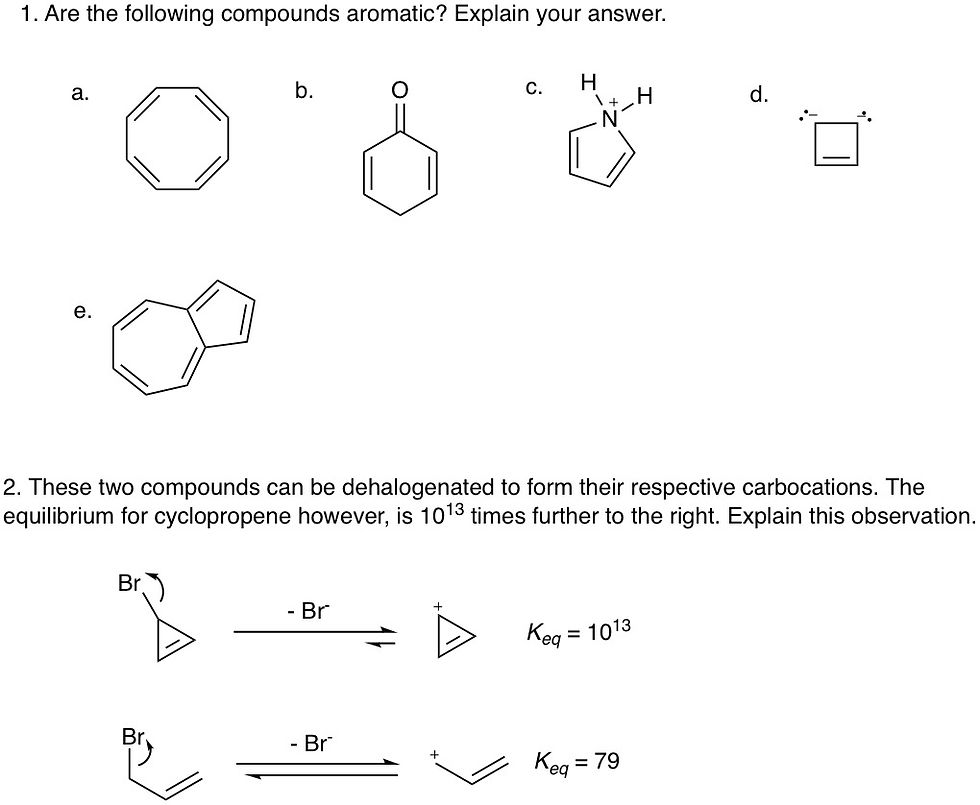
Comments