Chemical Equilibrium and Energy Diagrams decoded
- Benjamin Hui
- Jan 18, 2019
- 6 min read
As a young student of chemistry, I was often intimidated by those squiggly graphs containing all sorts of weird symbols. It took me quite a while to figure out what those symbols mean, and even longer to grasp the concepts they signified. These energy diagrams forced me to try to imagine the molecular world in my mind, in what feeble way it could, and eventually I started to understand them a little better; why they look the way they are. I made up my mind on one thing: to consider ALL reactions to be in a state of equilibrium between reactants and products.
Before going any further, I think it would be appropriate to dispel any misconceptions about the word equilibrium in chemistry. Simply put, it is a state of a chemical reaction in which the rate of formation of product(s) is the same as the rate of formation of the reactants(s) i.e. the rates of the forward and backward reactions are the same.
Consider the reaction:
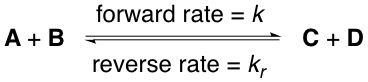
When A and B are mixed, they will react to form C and D, which will in turn react in some quantity to form back A and B and back and forth. Eventually this process comes to equilibrium, and the forward rate will be equal to the reverse rate i.e. k = kr. This does not mean that there will be 50% products and 50% reactants at equilibrium though! The ratio might be 70% products and 30% reactants at equilibrium, or 80-20, or something else. What equilibrium means is that the ratio of products to reactants stays constant. If we want to calculate this ratio, we can invoke a mathematical relation involving the Gibbs free energy, which I will explain later.
So remember what I mentioned about considering all reactions to be in equilibrium? Yes, that includes even those reactions that we were taught to be complete and irreversible, for example, a strong base reacting with strong acid, such as sodium hydroxide and hydrochloric acid. This neutralization reaction supposedly goes to completion to give ordinary table salt (sodium chloride) and water as products.
But that actually isn't the case. What it really means is that when this reaction reaches equilibrium, the ratio of products (NaCl and water) to reactants (HCl and NaOH) is so high that the reactants are considered negligible. Perhaps it is like 99.9999% products and only 0.0001% reactants. But since it's still an equilibrium, and if we had bionic eyes and were able to peer into the molecular realm and observe all the trillions upon trillions of molecules floating around, we would see the reverse reaction taking place here and there (NaCl and water reacting to form NaOH and HCl!), albeit extremely rarely! This is why we write this reaction with a unidirectional arrow. Using an equilibrium arrow would be pointless.
So why do most reactions not have 50% products and 50% reactants at equilibrium? First of all that would be super boring, and most of the chemistry that we have painstakingly developed over the centuries would not exist. Secondly, and most importantly, is that every single molecule has a different energy content. A molecule's energy is what fingerprints are to humans: a unique identifier of sorts. No two molecules have the same energy. It is this inequality in energy that allows molecules to be interconverted. And if a molecule is higher in energy, the more unstable it is, which makes sense: it would be like a hyperactive child, wanting to react with everything it can around it.
So, if a reaction had products that were lower in energy than the reactants, the equilibrium would tend to favour the formation of the products, which makes sense right? This is where we can now get to our energy diagrams. Let's take a look at a simple one:
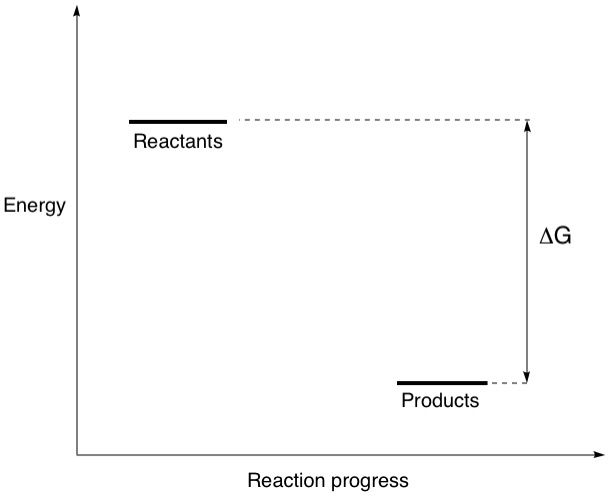
The diagram shows where the reactants and products are in terms of their energy content. The energy difference between them is called the Gibbs free energy, delta(G). Simply put, this is the energy released or absorbed when reactants change their molecular structures (by the breaking and forming of bonds) to become products. The magnitude and sign of delta(G) determines the ratio of products to reactants that we discussed earlier and is represented by the symbol K, the equilibrium constant. We can calculate K if delta(G) is known via the relation K = e^(-delta(G)/RT) where R is the ideal gas constant and T is the temperature. In the diagram above, the products are lower in energy than the reactants, meaning they are more stable. This means that this reaction is spontaneous, or exergonic. All spontaneous reactions have a larger proportion of products than reactants at equilibrium, reflecting a negative delta(G) value and a K value greater than 1.
Does being spontaneous mean that reactant molecules can decide on their own free will when to become products? The last time we checked, molecules aren't sentient and can only react when motivated, such as changing the external conditions. Keep in mind that molecules are physical objects that need to actually collide to react, and even so, need to collide with high enough energy to do so. Most of the time, this means providing a sufficiently high temperature. This external energy that needs to be inputted for the reaction to occur is called the activation energy and is represented by the symbol Ea. We can now add this to our energy diagram:
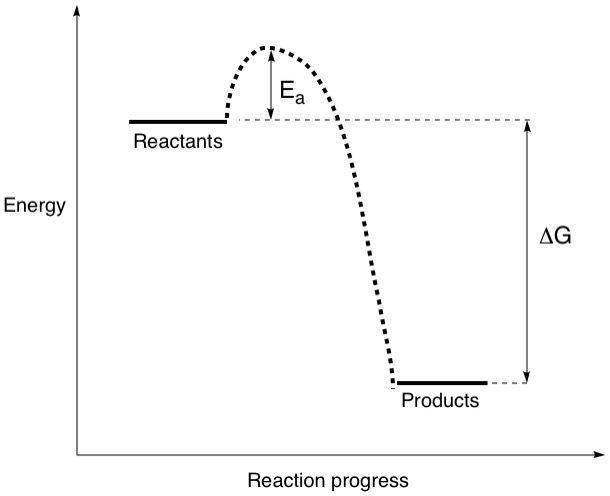
Imagine rolling a large stone up a hill. That requires you to exert energy. But when you get to the top, the stone will roll down the other side by itself, requiring no further energy input on your part. This is exactly the same as a spontaneous reaction. Once this activation energy barrier is overcome by providing the appropriate amount of energy, the reactants will react and become products.
Is there a situation where the products are higher in energy than the reactants? Absolutely. Let's take the same energy level diagram and swap the reactants and products.
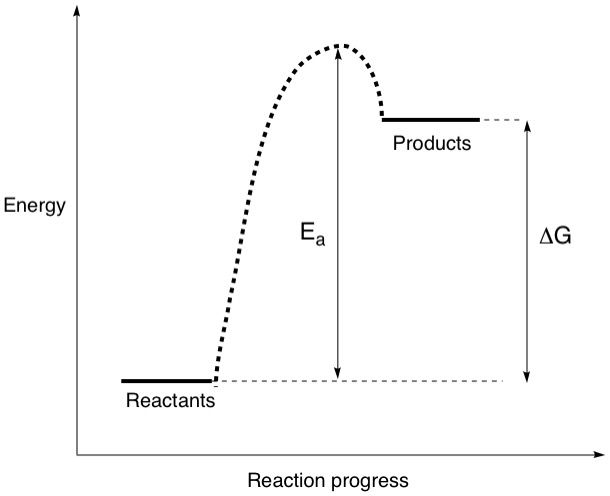
By doing this, two things have changed: First, the sign of delta(G) has changed, from negative to positive. The reaction is now non-spontaneous, or endergonic. This means that at equilibrium, there is a larger proportion of reactants than products, reflecting a positive delta(G) and a K value smaller than 1. Second, the activation energy has increased, by a lot! Pushing the rock up that hill just got a lot harder, and a lot more energy needs to be put in! This also means that it will take you a much longer time to get that rock to the top doesn't it? So, the larger the value of Ea, the slower the reaction gets i.e. the reaction rate decreases.
Your curious mind might be going, "But wait! What if the energy content of the reactants and products is roughly the same? Does that ever happen?" It sure does! This situation is pretty rare but it does exist. Let's call up the energy diagram again.
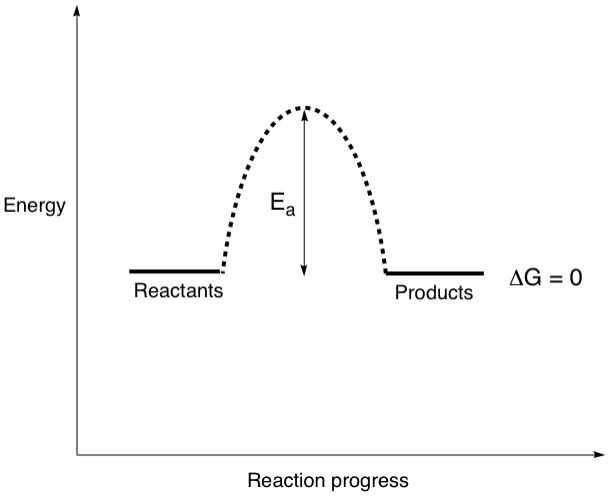
This time, delta(G) is zero. This would give a K value of 1 which means that at equilibrium, there will be 50% reactants and 50% products. Because of how rare this situation is, we chemists never bothered to come up with a special name for it. I've made up my own name for it, EQUALibrium!
You may be wondering, why is Ea measured from reactants to the top of that hill? Is there a special name for the hilltop? There sure is. It's called the transition state, and it is the instance of the reaction where old bonds are being broken and new bonds are being formed simultaneously, a result of the reactants colliding at the correct location, speed and geometry. The transition state is not a real molecular entity. Rather, it is simply the energy maximum of the reaction.
One final thing before I leave us to digest what we just learned. Remember how the value of Ea affects the rate of the reaction? Sometimes, we chemists know the value of a particular reaction and want to exploit it, but it is too slow to be of any practical use. Is there a way to speed up the reaction? Certainly, and that's where catalysts come into play. Catalysts are chemical substances that increase the rate of reactions by lowering the Ea. Let's have a final look at the energy diagram.
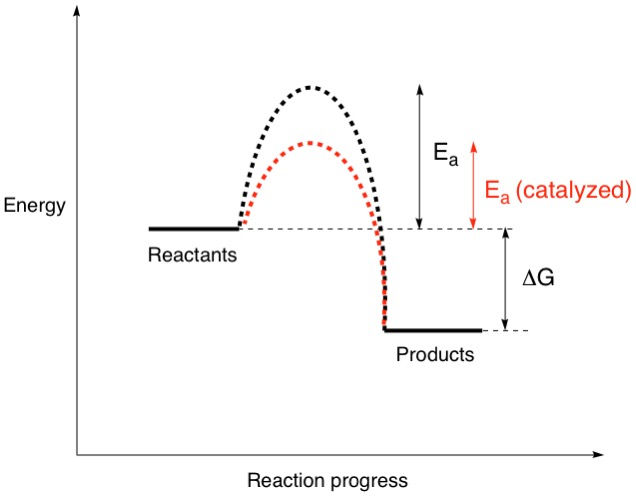
We see that the catalyzed reaction now has a lowered Ea. It's now easier to push that rock up the hill, and we could do it faster!
For a real life example of catalysis in action, all we have to do is remember those times when we played outside and got ourselves hurt, like getting badly cut. Mom would break out a bottle of hydrogen peroxide from the medicine cabinet and apply it on the cut, the hydrogen peroxide would bubble instantly, releasing oxygen to disinfect the wound. We probably never noticed this as kids because we were too busy screaming in pain. But if that hydrogen peroxide were left alone, it would decompose into water and oxygen only very slowly. The enzyme catalase in our blood lowers the Ea of this decomposition by so much that it increases its rate by many millions of times!
I have endeavoured to be as brief as possible in this article to explain chemical equilibrium and help students understand the concepts behind reaction energy diagrams. Do feel free to contact me if you have further questions or need further clarification.
Comments