Say you were given five Lego blocks, and were told to assemble something out of them. How many ways could you put them together? My guess would be that there's more than one. Each possible structure will have a unique arrangement of the blocks, and would have different three dimensional shapes. They would also have different properties. For example, a circular-shaped one would be able to roll across the floor to some extent, while a rectangular one wouldn't. Organic molecules aren't that much different than Lego sets, they're made up of blocks i.e. atoms that can be assembled in numerous ways! Molecules that have the same atomic makeup i.e. the same number of the same kinds of atoms, but with different arrangements of the atoms are called isomers. These are further subdivided into two categories: constitutional isomers and stereoisomers.
Constitutional isomers:
These are molecules that have different bond connectivities, whereby atoms, or groups of atoms (substituents), have changed location to a different part of the molecule. Here are a couple of examples.
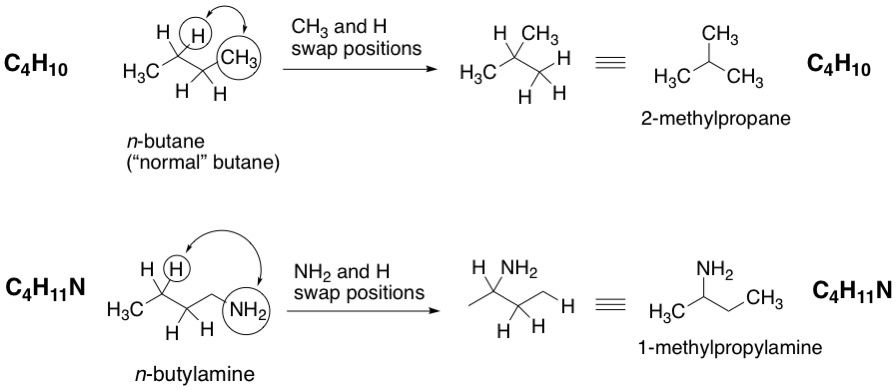
These molecules and their constitutional isomers are now structurally different (they can also be referred to as structural isomers), and so their physical properties are also different e.g. boiling points, polarities.
Stereoisomers:
Unlike constitutional isomers, stereoisomers differ in the their orientations of atoms in space. There are no positional swaps in stereoisomers. Atoms, or substituents, remain connected to where they were originally connected to. So wouldn't that make these molecules essentially identical? In a way, yes. They have identical physical properties such as boiling points and polarities. But they differ in one major aspect: chirality. This is a property that is actually a fundamental occurrence in nature. If you held both your palms up, and tried to overlay them, you'd find that it would be impossible to superimpose them. Snail shells are also chiral; you have both left-spiralling ones and right-spiralling ones. Likewise, they can't be superimposed on each other.
In molecules, two otherwise identical ones, but with different chirality will rotate plane-polarized light in equal magnitude, but different directions. For example, one molecule would rotate the light 30º to the left of vertical, while the other would rotate the light 30º to the right of vertical. They are otherwise indistinguishable with identical physical properties, and cannot be separated by conventional lab techniques such as chromatography. These two molecules would then be called enantiomers, and they must be non-superimposable mirror images of each other. How is this criteria met? They must contain a chiral center, which in most cases is a carbon atom bound to four different substituents. Let's look at an example.
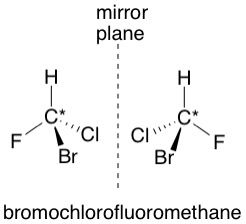
These two molecules of bromochlorofluoromethane are enantiomers, and you'll find that they can't be superimposed, no matter how they are manipulated (the asterisk at carbon denotes that it is a chiral center). The best way to see this is to build models of them. Molecular model kits are indispensable in organic chemistry, and everyone should have one!
How do we distinguish between enantiomers then? We assign priorities to each substituent based on a set of rules, called the Cahn-Ingold-Prelog (CIP) rules. I will only touch on one of these rules here, which is the assignment of priorities to each substituent based on their atomic weights. For a more comprehensive breakdown of the CIP rules, I recommend both the ChemLibre text and Master Organic Chemistry. Using the two examples of bromochlorofluoromethane above, we see that bromine is the heaviest atom, so it will be given first priority (1), followed by chlorine (2), fluorine (3) and lastly hydrogen (4). Next, the molecules need to be manipulated so that the lowest priority atom (H) is pointing away from you. This will make it eclipsed by the carbon. The direction of the circle traced out by the 1st, 2nd and 3rd priority atoms will determine the configuration of the enantiomer, either R or S. The following diagram explains this.
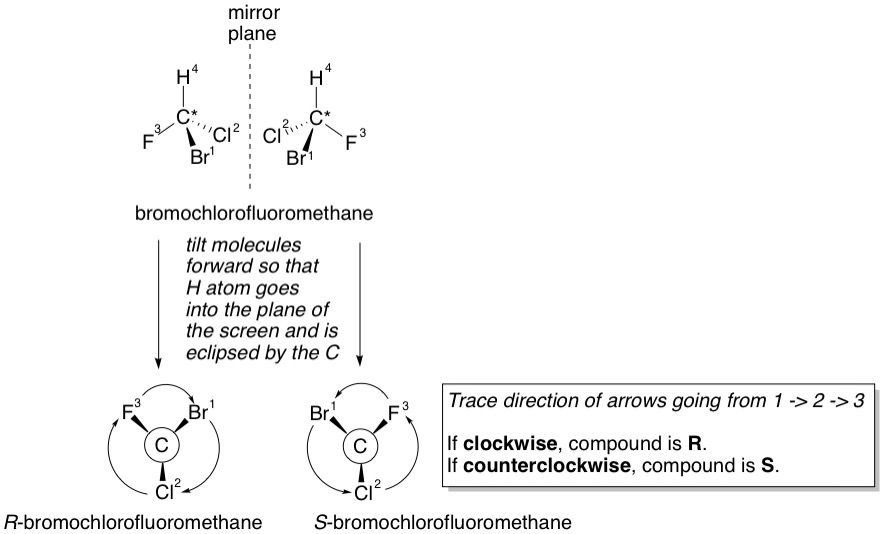
What if there is more than one chiral centre in a molecule? The same rule applies. You would figure out the configuration of each chiral centre. Once completed, you would simply flip the configurations of all the chiral centres so R becomes S or vice versa to get the corresponding enantiomer. The example below shows this.
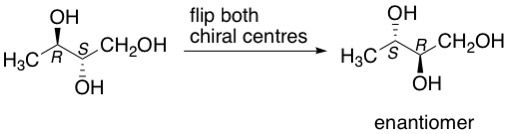
Enter the diastereomer:
What happens if you flip anything less than all the chiral centres in the molecule? These would not be enantiomers anymore, but diastereomers. With the above example, say you flipped only the S chiral centre to R, you would get its diastereomer. Similarly, if you flipped only the R chiral centre to S, you would get another diastereomer.
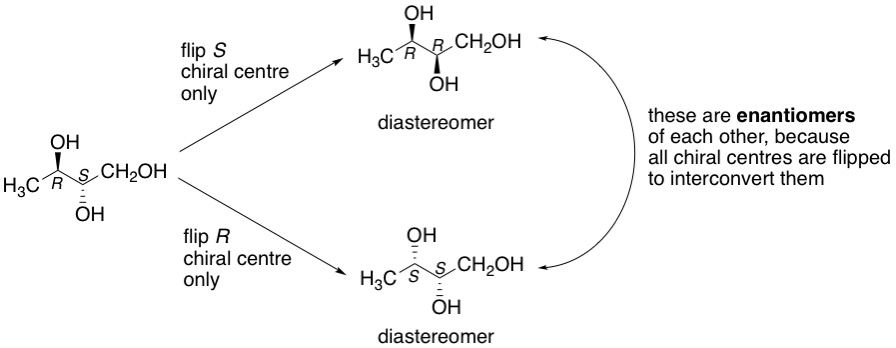
Diastereomers, unlike enantiomers, have different physical properties and can be separated by chromatography, because they are now no longer mirror images of the original compound.
Special kinds of diastereomers:
If flipping one chiral centre out of several in a molecule makes a diastereomer, do other situations analogous to this exist? Let's look at a double bond-containing molecule, such as an alkene like 2-butene. This molecule can exist in either cis, where the terminal methyl groups are on the same side, or trans where they are on opposite sides.
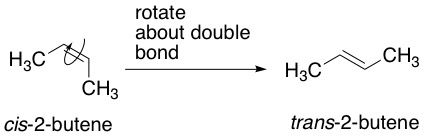
The net difference when converting between these two forms is the change in position of one of the methyl groups. Cis-trans isomerism in alkenes is therefore considered a type of diastereoisomerism, called geometric isomerism.
Another variant of diastereoisomerism occurs as conformational isomerism, whereby the free rotation about a single bond leads to different conformers, or rotamers. An example is shown below in the form of n-butane.

If we keep the left side constant and rotate the central single bond 180º, the result would be the methyl groups coming into close proximity with each other, which isn't favourable energetically because of their large size and therefore experiencing steric repulsion. This conformer thus has a higher energy than the left one. With both of these conformers having different energy levels, they are considered to be distinct from each other, and therefore they qualify as diastereomers. This also applies to geometric isomers, because the cis isomer always has a higher energy than the trans isomer, again due to steric effects.
For reference, the diagram below summarizes the types of isomers commonly encountered in organic chemistry.
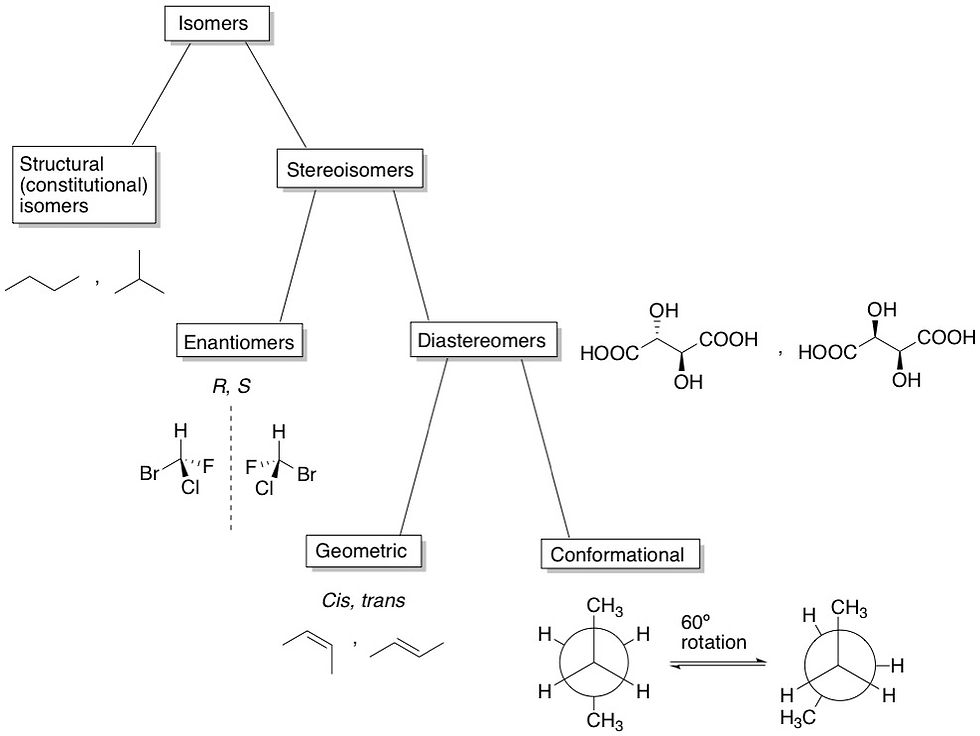
Comments